- Docente: Giorgio Baccarani
- Credits: 6
- SSD: ING-INF/01
- Language: Italian
- Teaching Mode: Traditional lectures
- Campus: Bologna
- Corso: Second cycle degree programme (LM) in Electronic Engineering (cod. 0934)
-
from Sep 16, 2024 to Dec 17, 2024
Learning outcomes
The aim of the course is to develop the required background to address the study of nanoscale semiconductor devices. At these dimensional limits, the occurrence of quantum mechanical effects related with carrier confinement becomes important, and the gate length turns out to be comparable, or smaller, than the carrier mean-free path. These effects require a re-examination of the physical models to be used for the interpretation and prediction of the device characteristics. The course is thus going to review the basic foundations of quantum mechanics, the theory of semiconductor physics, as well as the treatment of non-equilibrium transport in semiconductors. The mathematical model is thus based on the coupled Schroedinger and Poisson equations, to be solved with the boundary conditions characterizing the specific device geometry and morphology. Modern semiconductor devices will be studied using both the classical transport model based on drift-diffusion, as well as the quantum-mechanical model under the assumption of ballistic transport. The student will thus become aware of the limitations of the obtained solutions by comparing the different results provided by the two models.
Course contents
Introduction
The evolution of the microelectronic technologies has led to the fabrication of integrated systems containing some billions of elementary transistors with linear dimensions of the order of ten nanometers which, in many cases, are smaller than, or comparable with, the electron mean-free path. The miniaturization process of electron devices has thus reached a level which makes the classical treatment of carrier transport in semiconductors no longer adequate. The drift-diffusion transport model is in fact based on the opposite assumption, namely that the electric field within the device changes over a space scale much larger than the carrier mean-free path, and over a time scale much longer than the average time between collisions. Also, the structural confinement of the carriers is responsible for novel quantum-mechanical effects, such as energy quantization and, thus, the splitting of the conduction and valence bands into a multiplicity of sub-bands with a smaller dimensionality. Finally, direct source-to-drain and band-to-band tunneling effects are going to play an ever increasing role. In view of the previous considerations, a re-examination of the classical methodologies currently used for the analysis of electron devices becomes mandatory.
The course of Nanoelectronics aims to address such a need, and to investigate the properties of carrier transport in nanometric-scale structures, also referred to as mesoscopic systems. This term means that these systems are still large with respect to the atomic dimensions, but, at the same time, smaller or comparable with the electron mean-free path. The concept of local quasi-equilibrium is thus abandoned and so is the description of carrier transport via the concepts of mobility and diffusivity. The nature of the new constitutive equations becomes strongly non-local and the importance of the boundary conditions, by which the device under investigation is isolated from the neighboring circuit, increases.
The devices to be studied are going to include, due to their practical importance, tri-gate (TG) FETs, namely, the basic components of technology nodes from 22 nm down to 2 nm; fully-depleted (FD) silicon-on-insulator (SOI) transistors, silicon nanowire field-effect transistors (NW-FETs) and multiple nanosheet transistors (NS-FETs). The course will additionally examine homo- and heterostructure band-to-band tunneling transistors (T-FETs), the interest of which is related to their steep subthreshold slope, and transistors based on two-dimensional materials, including graphene and transition-metal dichalcogenide materials.
Nanoelectronics Course Content
Structure of matter
- Historical background
- Review of principles of Quantum Mechanics
- Elements of Quantum Electrodynamics
- Elementary applications: the hydrogen atoms
- Electron spin: Pauli equations
- Fundamentals of relativistic Quantum Mechanics. Dirac equation
- Time dependent and independent perturbation theory. The WKB method.
Particle systems and solid-state Physics
- Electron indistinguishability principle
- Adiabatic approximation (Born-Oppenheimer)
- Lattice vibrations in molecules and solid bodies
- Quantum statistics
- Electrons and holes in semiconductors
- Bloch Theorem
- Effective-mass theorem
- Energy bands in semiconductors
Carrier transport in semiconductors
- The Boltzmann equation
- The continuity equation in semiconductor
- Classical transport equations in semiconductors
Nanoscale-size devices
- Double-gate symmetric transistor (FinFET like)
- Fully depleted silicon on insulator transistor (FD-SOI FET)
- Surrounding-gate nanowire transistor (NW-FET)
- Surrounding-gate nanosheet transistor (NS-FET)
- Band-to-band tunnel transistor (T-FET)
- General properties of 2D material-based transistors
Readings/Bibliography
S. Datta: "Electronic Transport in Mesoscopic Systems", Cambridge University Press
S. Datta: "Quantum Transport: Atom to Transistor", Cambridge University Press
D. H. Ferry, S. M. Goodnick: "Transport in Nanostructures", Cambridge University Press
M. Lundstrom: "Fundamentals of Carrier Transport", Cambridge University Press
L. I. Schiff: "Quantum Mechanics", McGrow-Hill
M. Rudan: "Physics of Semiconductor Devices", Springer
Teaching methods
Traditional lectures are delivered in the classroom, illustrating the most important physical concepts of the discipline. The required calculations leading to the main results are carried out at the blackboard. Occasionally, slides are used for a better presentation of images, not otherwise reproducible on the blackboard.
Assessment methods
The assessment of the student learning will occur via oral examination.
Teaching tools
A number of textbooks are suggested for consultation, and personal notes of the teacher are delivered to the students.
Office hours
See the website of Giorgio Baccarani
SDGs
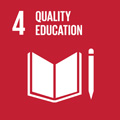
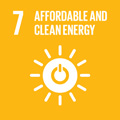
This teaching activity contributes to the achievement of the Sustainable Development Goals of the UN 2030 Agenda.